Collagen is the most abundant protein in the body. It provides structure, support, & strength to the skin, muscles, bones & connective tissue. It is what keeps the skin plump, firm, & youthful looking. As we age, the body’s collagen production starts to decline. Stress, pollution & sun exposure are some of the major factors that affect collagen production. During menopausal years, women’s estrogen levels decline which causes a dramatic loss of collagen, as much as 30%. BIOKOR Collagen is an advanced collagen formula with 5 Types of Collagen, enriched with Biotin, Hyaluronic Acid and Vitamin C which helps & supports the body’s collagen production.
TYPES OF COLLAGEN
Type 1 collagen is the most abundant collagen in the body. It is densely packed and used to provide structure to your skin, bones, tendons and ligaments. It is the most instrumental in promoting skin health.
Type 1 collagen makes up about 90% of the body’s collagen. However, an individual can lose collagen components in the body due to exposure to ultraviolet light, tobacco, excessive intake of sugar, and aging. This loss of collagen can cause the skin to lose elasticity, reduction of the thickness of the epidermis, increase in the formation of wrinkles and sagging, and also make the skin vulnerable and easily damaged.
Type II collagen is the main component and key structural component of cartilage. It makes up 50% of all protein in cartilage and 85–90% of collagen of articular cartilage. It is what gives cartilage strength and elasticity. Having adequate levels of collagen is essential for keeping joints healthy and flexible.
Type 3 collagen is found in muscles, arteries and organs. It is the second most abundant collagen type in your body but declines drastically with age. It complements and works alongside type 1 Collagen. It regulates type 1 collagen fibril formation, and can be found in reticular fibers such as bone marrow.
Type 5 collagen is a fibrillation collagen and is an abundant protein in skin, placenta and lung, and essential for tissue elasticity and compliance.
Type 5 collagen is essential for fibrillation of types I and III collagen, and consequently for optimal fibrillary formation and tissue quality.
Type V collagen contributes to the bone matrix, corneal stroma, and the interstitial matrix of muscles, liver, lungs, and placenta.
Type X collagen is a reliable marker for new bone formation in articular cartilage.
Articular cartilage is the smooth, white tissue that covers the ends of bones where they come together to form joints. Healthy cartilage in our joints makes it easier to move. It allows the bones to glide over each other with very little friction.
Eggshell membrane helps maintain joint and cartilage health. It is essential in promoting Collagen type V and X production.
Eggshell membrane collagen also gives your skin its firmness. It supports the barrier function of your skin, which is what protects your body from outside invaders such as bacteria and viruses.
Eggshell membrane has demonstrated efficacy in relieving joint pain and stiffness. It is composed mainly of fibrous collagen proteins, types I, V, and X. It also contains glycosaminoglycans, such as chondroitin sulphate and dermatan sulphate, and hexosamines, such as glucosamine.
In addition, hyaluronic acid has been shown to be present in significant amounts.
Biotin, also known as Vitamin B7, stimulates keratin production & helps increase the rate of follicle growth resulting in thicker and healthier hair and stronger nails. It also improves skin hydration and smoothness.
Hyaluronic acid is an essential component of the skin because of its ability to promote collagen. It is found naturally in the skin, where it binds water to retain moisture. According to Clinical Cosmetic Investigational Dermatology’s study, Hyaluronic acid inhibits skin wrinkles and improves overall skin condition.
Vitamin C is not only a powerful antioxidant which boosts the immune system. It also helps prevent premature skin aging and delays the signs of biological skin aging. Vitamin C increases and stimulates the body’s collagen production resulting to healthier, younger looking and radiant skin.
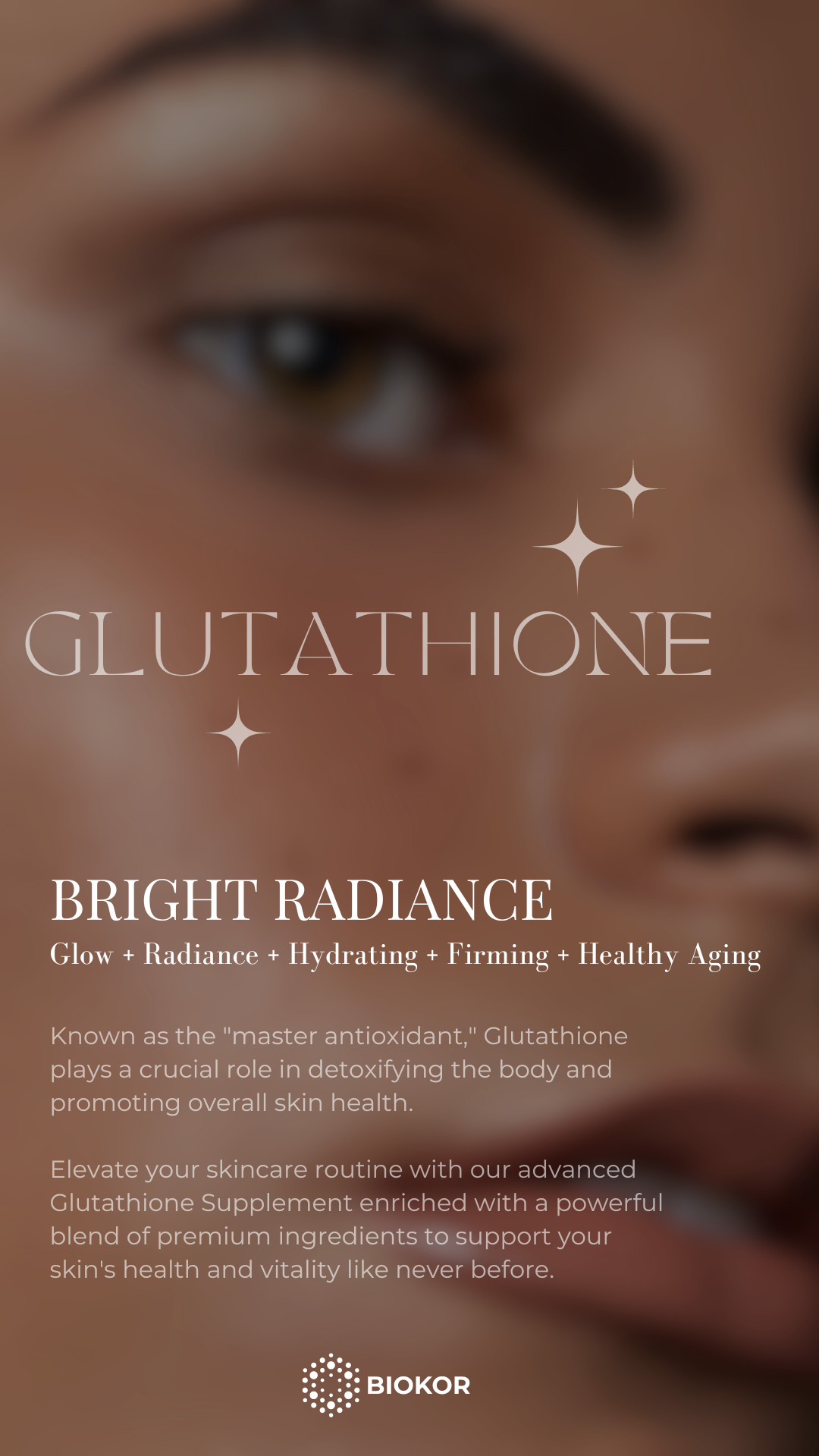
Glutathione, considered to be the master antioxidant (AO), is the most-important redox regulator that controls inflammatory processes, and thus damage to the periodontium.
“Antioxidants (AOs) are molecules, which when present at low concentrations compared to those of an oxidizable substrate, will significantly delay or inhibit oxidation of that substance.”[3,4]
Oxidative stress (OS) leads to molecular damage resulting from disturbances in redox signaling and control due to an imbalance between oxidants and AOs oxidants ROS.[10,11,12] Oxidative Stress is considered to play an important role in the pathogenesis of chronic inflammatory conditions like non-insulin dependent diabetes mellitus, cardiovascular disease, metabolic syndrome,[11,13,14,15] acute respiratory distress syndrome,[5,16] and periodontal disease.[5,17,18,19,20,21,22] Recently, it has been proposed as a central link between systemic diseases and periodontitis.[23]
Ubiquitous Antioxidant master, glutathione is a low-molecular weight, major non-protein cellular thiol, present in both eukaryotic as well as prokaryotic cells,[24,25,26] and exist in every single cell of the human body.[24]
It is a potent Antioxidant that prevents ROS mediated damage to essential cellular components and acts as a cofactor for enzymes in the destruction of ROS. It serves as a reservoir for cysteine, participates in detoxification reactions for xenobiotics and metabolism of numerous cellular compounds (e.g., NO), and is required for synthesis of some prostaglandins (PGs) and thermotolerance.[25] It also has a significant role in nutrient metabolism and regulating cellular events as cell apoptosis, cell proliferation, cytokine production, immune response, gene expression, DNA and protein synthesis, signal transduction and protein glutathionylation.[27]
Background
Previous studies showed that supplementation of reduced form of glutathione (GSH, 500 mg/d) has a skin-lightening efficacy in humans. This study was designed to evaluate the influences of both GSH and oxidized form (GSSG), at doses lower than 500 mg/d, on improving skin properties.
Patients and methods
A randomized, double-blind, placebo-controlled, parallel, three-arm study was conducted. Healthy female subjects were equally randomized into three groups and took GSH (250 mg/d), GSSG (250 mg/d), or placebo orally for 12 weeks. At each visit at baseline and for 12 weeks, skin features including melanin index, wrinkles, and other relevant biophysical properties were measured. Blood samples were collected for safety monitoring.
Results
In generalized estimating equation analyses, melanin index and ultraviolet spots of all sites including face and arm when given GSH and GSSG tended to be lower than placebo. At some sites evaluated, subjects who received GSH showed a significant reduction in wrinkles compared with those taking placebo. A tendency toward increased skin elasticity was observed in GSH and GSSG compared with placebo. There were no serious adverse effects throughout the study.
Conclusion
We showed that oral glutathione, 250 mg/d, in both reduced and oxidized forms effectively influences skin properties. Overall, glutathione in both forms are well tolerated.
In skin, ASX has been shown to improve dermal health by direct and downstream influences at several different steps of the oxidative stress cascade, while inhibiting inflammatory mediators at the same time [12]. Molecular and morphological changes in aged skin not only compromise its protective role, but also contribute to the appearance of skin symptoms, including excessive dryness and pruritus, as well as increased predisposition to the formation or deepening of wrinkles, dyspigmentation, fragility and difficulty in healing injuries, alteration in skin permeability to drugs, impaired ability to sense and respond to mechanical stimuli, skin irritation, and tumor incidence [13,14]. The effects of ASX on hyperpigmentation suppression, melanin synthesis and photoaging inhibition, and wrinkle formation reduction have been reported in several clinical studies [15].
In the current review, we will address some issues that highlight the overall versatility and protection offered by ASX. In particular, we will discuss the effects of ASX on cellular and molecular mechanisms, such as the regulation of antioxidant and anti-inflammatory activities, modulation of the immune response, prevention of skin damage, and regulation of DNA repair.
Antioxidant Activity
Oxidative stress plays a crucial role in human skin aging and dermal damage. The mechanisms of intrinsic (chronological) and extrinsic (photo-) aging include the generation of reactive oxygen species (ROS) via oxidative metabolism and exposure to sun ultraviolet (UV) light, respectively. Thus, the formation of ROS is a pivotal mechanism leading to skin aging. Oxidant events of skin aging involve damage to DNA, the inflammatory response, reduced production of antioxidants, and the generation of matrix metalloproteinases (MMPs) that degrade collagen and elastin in the dermal skin layer [16,17,18]. There are many dietary or exogenous sources that act as antioxidants, including polyphenols and carotenoids [19,20]. ASX has recently caught the interest of researchers because of its powerful antioxidant activity and its unique molecular and biochemical messenger properties with implications in treating and preventing skin disease. Comparative studies examining the photoprotective effects of carotenoids have demonstrated that ASX is a superior antioxidant, having greater antioxidant capacity than canthaxanthin and β-carotene in human dermal fibroblasts. In particular, ASX inhibits ROS formation and modulates the expression of oxidative stress-responsive enzymes such as heme oxygenase-1 (HO-1), which is a marker of oxidative stress and a regulatory mechanism involved in the cell adaptation against oxidative damage [21].
Anti-Inflammatory Properties
Extensive research during the last two decades has revealed the mechanism by which continued oxidative stress leads to chronic inflammation, which in turn, mediates most chronic diseases including neurodegeneration, cancer, and skin damage [27,28,29]. It is well established that various proinflammatory markers in skin are increased as a result of UV exposure. Keratinocytes play a crucial role in the photodamage response after UV exposure by releasing proinflammatory mediators. It has been shown that ASX treatment prevents the deleterious effects of UV by decreasing UV-induced reactive nitrogen species production, inflammatory cytokine expression, and apoptosis in keratinocytes.
Immune-Enhancing Effects
Considerable evidence suggests that suppression of immune system contributes to the development of solar UV-induced cutaneous malignancies, including melanoma and non-melanoma, in both mouse models and humans [34,35,36]. Astaxanthin significantly influences immune function in several in vitro and in vivo assays [37]. For example, in vitro studies on human lymphocytes have demonstrated enhancement by Astaxanthin of immunoglobulin production in response to T cell-dependent stimuli [38].
In these studies, Astaxanthin increased natural killer (NK) cell cytotoxic activity, suggesting that Astaxanthin may regulate NK cells that serve as an immunosurveillance system against tumors and virus-infected cells [39,40]. Moreover, other authors have shown that Astaxanthin increased cytotoxic T lymphocyte activity in mice. Activated T cells and NK cells produce interferon-γ (IFN-γ), which is involved in immune regulation and B cell differentiation; therefore, Astaxanthin may enhance immune responses and potentially exert antitumor activity [41].
Effects on Skin Damage
The most important and abundant structures of the dermal extracellular matrix (ECM) are collagen, elastin, and glycosaminoglycans (GAGs). In both intrinsic and extrinsic aging, changes in these structures are observed. These modifications lead to the loss of tensile strength and recoil capacity, wrinkle formation, dryness, and impaired wound healing [45]. In addition, UV-induced ROS stimulate the synthesis of MMPs that are responsible for the degradation of ECM, and in particular, MMPs can fully degrade collagen [46]. In vitro, Astaxanthin effectively suppresses cell damage caused by free radicals and induction of MMP-1 in skin after UV irradiation [47]. Some similar studies also reported that Astaxanthin inhibited the expression of MMPs in different cells, including macrophages and chondrocytes [48,49]. Recently, an enriched Astaxanthin extract from H. pluvialisincreased collagen content through inhibition of MMP-1 and MMP-3 expression in human dermal fibroblasts [50]. It has been shown that Astaxanthin is an effective compound for accelerating wound healing in full-thickness dermal wounds in mice. Astaxanthin treated wounds showed significantly increased expression of wound healing biological markers such as collagen type I α 1 (Col1A1) and basic fibroblast growth factor (bFGF) [52].
Evidence from Human Clinical Trials
Both in vivo and in vitro studies have demonstrated that Astaxanthin may play a promising functional role to treat and prevent skin aging.
The potential skin-protective effects of Astaxanthin have also been investigated in humans. The main source of Astaxanthin intake in humans is from seafood, with wild sockeye salmon, for example, providing 26–38 mg/kg of flesh [61].
In 2001, Seki et al. [67] conducted a small pilot study with ASX from H. pluvialis to investigate the wrinkle reduction effect on the skin of 45 healthy subjects. The authors observed an antiwrinkle effect in female human subjects (n = 3), using a topical cream containing ASX combined with other active ingredients. A dermatological assessment revealed significant reduction of wrinkles and puffiness on the lower eye and cheeks after two weeks of use.
A second preliminary human study performed by Yamahita in 1995 [68] showed in healthy male subjects (n = 7) that topical natural ASX from krill significantly reduces erythema by 60% at 98 h after UV-B exposure.
In a second study, the same author administered to 49 healthy female subjects (mean age of 47 years) 2 mg of Astaxanthin or placebo. After six weeks of treatment, significant improvements were observed in skin moisture and elasticity [69].
In another study by Tominaga et al. [70], the effect of Astaxanthin on wrinkle reduction and skin elasticity was investigated in 28 female subjects (20–55 years). The combined use of a dietary supplement and a topical product containing Astaxanthin for eight weeks showed a reduction in the overall average wrinkle depth.
Several studies demonstrated that the combined administration of Astaxanthin with other compounds, particularly collagen hydrolysate, may show additive or synergistic effects for preventing or reversing the skin aging process [75,76]. Consistent with this, a recent study with 44 healthy subjects showed that a combination of Astaxanthin 2 mg/day) and collagen hydrolysate (2 mg/day) for 12 weeks improves elasticity and barrier integrity in human skin.
In an open-label noncontrolled study, 30 healthy female subjects received for eight weeks 6 mg per day of oral supplementation combined with 2 mL (78.9-μM solution) per day of a topical application of ASX. Significant improvements were observed in skin wrinkle, age spot size, elasticity, and skin texture [15]. The same authors also conducted a randomized double-blind placebo-controlled study involving 36 healthy male subjects supplemented with 6 mg of Astaxanthin for six weeks. At the end of the study period, Astaxanthin improved wrinkles, elasticity, transepidermal water loss (TEWL), moisture content, and sebum oil level [15].These results demonstrate that Astaxanthin may improve skin condition in both men and women. Further evidence from human intervention studies is required. In addition, we recommend additional research focused on stimulation of the endogenous antioxidant defense systems of the skin, particularly the expression of antioxidant responsive elements associated with the activity of detoxifying enzymes.
Resveratrol, a compound belonging to polyphenols, besides its action on the cardiovascular system, affects also wound healing, regeneration, and photoaging of the skin. By interactions with numerous substances and pathways, e.g. MAPK, MAPKK, FOXO3, TGF or metalloproteinase 1, it protects the skin against the harmful effects of type B ultraviolet radiation, which is the main factor in the skin aging processes. It also enhances collagen synthesis by activating the oestrogen receptor and reduces wrinkles. In damaged tissues, it accelerates skin regeneration and healing by activating, among others, VEGF. Based on the review of the literature, there is no doubt that resveratrol has the potential to be used in cosmetology, dermatology and plastic surgery. It can be used as a compound of anti-aging products or as a topical treatment of scars and wounds. In the future this polyphenol might be applied in pharmacotherapy of many dermatoses.
The widespread use of resveratrol, in addition to its cardioprotective or neuroprotective effects, also includes skin regeneration, accelerated wound healing and prevention of skin aging caused mainly by type B ultraviolet radiation (Figure 2). Several studies confirming this effect are available, both on an animal and a human model (Table 2). This gives hope that resveratrol will be widely used in cosmetology as an anti-aging agent or in many medical disciplines such as surgery or dermatology as a healing accelerator.
Subedi et al. in 2017 analysed the effect of resveratrol derived from rice on skin exposed to type B ultraviolet radiation at 144 mJ/cm2. Polyphenol slowed the skin’s photoaging processes, including wrinkle reduction by inhibiting the caspase-3-cytochrome C and p-53-Bax complex pathway and the MMP-1 pathway. Reduced inflammation was also observed, due to inhibition of COX-2, interleukin 6, tumour necrosis factor-α (TNF-α) p38 mitogen-activated protein kinases, nitric oxide synthesis or c-Jun N-terminal kinases as well as prevention of decrease in elastin, procollagen 1 and transforming growth factor-β (TGF-β) [20].
A similar study was carried out by Lee et al. They administered resveratrol (in pure form or as a rice component) on human UV-B irradiated fibroblasts. It was found that compared to the control group, the skin treated with polyphenol had a higher content of procollagen 1, while lower activity of metalloproteinase 1 [21].
Resveratrol also stimulates the production of auto-phagocytes, which are responsible for the elimination of abnormal cells in the body, including the skin. As a result of the supply of polyphenol, cells from the HaCaT line showed a higher concentration of LC3 – II (light chain 3, a protein important for the process of autophagocytosis), as a result of transformations from LC3 – I. Increased LC3 breakdown resulting from lysosome fusion was also observed [23].
Pomegranate (Punica granatum L.), commonly known as a seeded or granular apple, is a delectable fruit eaten worldwide. Pomegranate is one of the healthiest fruits, with a high concentration of phenolic compounds.
PoP is a rich source of polyphenols including phenolic acids, tannins, and flavonoids, especially anthocyanin. These peels offer several functional and nutraceutical qualities owing to their bioactive ingredients, including lowering blood pressure, reducing oxidative stress, lowering cholesterol levels, and restoring heart health.
Skin aging is a multifactorial process consisting of two distinct and independent mechanisms: intrinsic and extrinsic aging. Youthful skin retains its turgor, resilience and pliability, among others, due to its high content of water. Daily external injury, in addition to the normal process of aging, causes loss of moisture. The key molecule involved in skin moisture is hyaluronic acid (HA) that has unique capacity in retaining water. There are multiple sites for the control of hyaluronic acid (HA) synthesis, deposition, cell and protein association and degradation, reflecting the complexity of hyaluronic acid (HA) metabolism. The enzymes that synthesize or catabolize HA and HA receptors responsible for many of the functions of HA are all multigene families with distinct patterns of tissue expression. Understanding the metabolism of HA in the different layers of the skin and the interactions of HA with other skin components will facilitate the ability to modulate skin moisture in a rational manner.
Skin aging
Human skin aging is a complex biological process, not yet fully understood. It is the result of two biologically independent processes. The first is intrinsic or innate aging, an unpreventable process, which affects the skin in the same pattern as it affects all internal organs. The second is extrinsic aging, which is the result of exposure to external factors, mainly ultraviolet (UV) irradiation, that is also referred to as photoaging.1 Intrinsic skin aging is influenced by hormonal changes that occur with age,2 such as the gradual decreased production of sex hormones from the mid-twenties and the diminution of estrogens and progesterone associated with menopause. It is well established that the deficiency in estrogens and androgens results in collagen degradation, dryness, loss of elasticity, epidermal atrophy and wrinkling of the skin.3
Even though intrinsic and extrinsic skin aging are distinctive processes, they share similarities in molecular mechanisms. For example, reactive oxygen species (ROS), arising from oxidative cell metabolism, play a major role in both processes.4ROS in extrinsic or intrinsic skin aging induce the transcription factor c-Jun via mitogen-activated protein kinases (MAPK), leading to overexpression of matrix metalloproteinase (MMP)-1, MMP-3 and MMP-9 and prevention of the expression of procollagen-1.5 Therefore, elevated levels of degraded collagen and reduced collagen synthesis are pathologies occurring in intrinsically aged as well as photoaged skin.
Skin aging is also associated with loss of skin moisture. The key molecule involved in skin moisture is hyaluronan or hyaluronic acid (HA), a glycosaminoglycan (GAG) with a unique capacity to bind and retain water molecules.6 HA belongs to the extracellular matrix (ECM) molecules. During the past decades the constituents of the skin have been well characterized. In the beginning, most of the studies focused on the cells that comprise the skin layers, such as the epidermis, the dermis and the underlying subcutis. Recently, it is appreciated that ECM molecules that lie between cells, in addition to providing a constructive framework, they exert major effects on cellular function. These ECM molecules, although they appear amorphous by light microscopy, they form a highly organized structure, comprising mainly of GAG, proteoglycans, growth factors and structural proteins such as collagens. Yet, the predominant component of the skin ECM is HA.
Recent reviews have described the involvement of HA with respect to its role in angiogenesis,7 reactive oxygen species,8 chondrocytes,9 cancer,10,11lung injury,12,13 immune regulation14,15 and skin.16 This review presents in brief recent knowledge in HA biology and function and focuses on its involvement in skin aging.
Chemistry and physicochemical properties
HA is a non-sulphated GAG and is composed of repeating polymeric disaccharides of D-glucuronic acid and N-acetyl-D-glucosamine linked by a glucuronidic β (1→3) bond.17,18 In aqueous solutions HA forms specific stable tertiary structures.19 Despite the simplicity in its composition, without variations in its sugar composition or without branching points, HA has a variety of physicochemical properties. HA polymers occur in a vast number of configurations and shapes, depending on their size, salt concentration, pH, and associated cations.20 Unlike other GAG, HA is not covalently attached to a protein core, but it may form aggregates with proteoglycans.21 HA encompasses a large volume of water giving solutions high viscosity, even at low concentrations.13
Tissue and cell distribution of HA
HA is widely distributed, from prokaryotic,22,23 to eukaryotic cells.24 In humans, HA is most abundant in the skin,25-29 accounting for 50% of the total body HA,30 the vitreous of the eye,31 the umbilical cord,17 and synovial fluid,32,33 but it is also present in all tissues and fluids of the body, such as skeletal tissues,27 heart valves,34 the lung,35-39 the aorta,40 the prostate,41 tunica albuginea, corpora cavernosa and corpus spongiosum of the penis.42 HA is produced primarily by mesenchymal cells but also by other cell types.34-38,43
Biological function of HA
Over the past 2 decades there was considerable evidence presented that unraveled the functional role of HA in molecular mechanisms and indicated the potential role of HA for the development of novel therapeutic strategies for many diseases.
Functions of HA include the following: hydration, lubrication of joints, a space filling capacity, and the framework through which cells migrate.34 The synthesis of HA increases during tissue injury and wound healing25,44,45 and HA regulates several aspects of tissue repair, including activation of inflammatory cells to enhance immune response46-48 and the response to injury of fibroblasts49,50 and epithelial cells.51-55 HA also provides the framework for blood vessel formation7,45 and fibroblast migration,56,57 that may be involved in tumor progression.58 The correlation of HA levels on the cell surface of cancer cells with the aggressiveness of tumors has also been reported.59
The size of hyaluronic acid (HA) appears to be of critical importance for its various functions described above. HA of high molecular size, usually in excess of 1,000 kDa, is present in intact tissues and is antiangiogenic and immunosuppressive, whereas smaller polymers of HA are distress signals and potent inducers of inflammation and angiogenesis.38,46,60-63
Hyaluronic acid and skin aging
The most dramatic histochemical change observed in senescent skin is the marked disappearance of epidermal hyaluronic acid (HA), while HA is still present in the dermis.92 The reasons for this change in hyaluronic acid (HA) homeostasis with aging is unknown. As mentioned above, the synthesis of epidermal HA is influenced by the underlying dermis and is under separate controls from the synthesis of dermal HA.16,98 Progressive reduction of the size of the HA polymers in skin as a result of aging has also been reported.109 Thus, the epidermis loses the principle molecule responsible for binding and retaining water molecules, resulting in loss of skin moisture. In the dermis, the major age-related change is the increasing avidity of HA with tissue structures with the concomitant loss of HA extractability. This parallels the progressive cross-linking of collagen and the steady loss of collagen extractability with age.16 All of the above age related phenomena contribute to the apparent dehydration, atrophy and loss of elasticity that characterizes aged skin.
Premature aging of skin is the result of repeated and extended exposure to UV radiation.110,111Approximately 80% of facial skin aging is attributed to UV-exposure.112 UV radiation damage causes initially a mild form of wound healing and is associated at first with an increase of dermal HA. As little as 5 min of UV exposure in nude mice caused enhanced deposition of HA, indicating that UV radiation induced skin damage is an extremely rapid event.16 The initial redness of the skin following exposure to UV radiation may be due to a mild edematous reaction induced by the enhanced HA deposition and histamine release. Repeated and extensive exposures to UV ultimately simulate a typical wound healing response with deposition of scarlike type I collagen, rather than the usual types I and III collagen mixture that gives skin resilience and pliability.16
Alpha-lipoic acid is an organic, sulfate-based compound produced by plants, humans, and animals. As a potent antioxidant and a natural dithiol compound, it performs a crucial role in mitochondrial bioenergetic reactions. A healthy human body, on the other hand, can synthesize enough α-lipoic acid to scavenge reactive oxygen species and increase endogenous antioxidants; however, the amount of α-lipoic acid inside the body decreases significantly with age, resulting in endothelial dysfunction. Molecular orbital energy and spin density analysis indicate that the sulfhydryl (-SH) group of molecules has the greatest electron donating activity, which would be responsible for the antioxidant potential and free radical scavenging activity. α-Lipoic acid acts as a chelating agent for metal ions, a quenching agent for reactive oxygen species, and a reducing agent for the oxidized form of glutathione and vitamins C and E. α-Lipoic acid enantiomers and its reduced form have antioxidant, cognitive, cardiovascular, detoxifying, anti-aging, dietary supplement, anti-cancer, neuroprotective, antimicrobial, and anti-inflammatory properties. α-Lipoic acid has cytotoxic and antiproliferative effects on several cancers, including polycystic ovarian syndrome. It also has usefulness in the context of female and male infertility. Although α-lipoic acid has numerous clinical applications, the majority of them stem from its antioxidant properties; however, its bioavailability in its pure form is low (approximately 30%). However, nanoformulations have shown promise in this regard. The proton affinity and electron donating activity, as a redox-active agent, would be responsible for the antioxidant potential and free radical scavenging activity of the molecule.
As a short-chain fatty acid, α-lipoic acid is synthesized inside the human body to work as an antioxidant, safeguarding body cells from injury, and helping restore the scales of other antioxidants, like vitamins C and E (Moura et al. 2021). Several studies have shown that combining α-lipoic acid with fructose can reduce fructose-induced inflammation, hepatic oxidative stress, and insulin resistance. It is also found that α-lipoic acid can act as a chemopreventive agent because it inhibits the inflammation linked to carcinogenesis (Moon 2016). α-Lipoic acid can reduce inflammatory markers in patients with heart disease, as oxidative stress is assumed to be the main cause of many cardiovascular diseases, together with hypertension, and heart failure. Oxidative stress increases during the aging process, resulting in either enhanced ROS generation or diminished antioxidant safeguards. The incidence of cardiac disease is directly related to one’s age. Aging is also related to oxidative stress, which in turn leads to hastened cellular senescence and organ dysfunction.
Coenzyme Q10 (CoQ10) is a well-known antioxidant and serves as an essential carrier for electron transport and proton translocation in the mitochondrial respiratory chain. CoQ10 has been widely commercially available in Japan as a dietary and health supplement since 2001 and it is used for the prevention of lifestyle-related diseases induced by aging. Recently, it was stated that for Japan, which is facing an aging society, CoQ10 has been used in many skincare products. However, the physiological actions of CoQ10 in skin fibroblasts are not fully understood. In this study, we examined the effect of CoQ10 on cultured human skin fibroblast. In this study, CoQ10treatment increased intracellular CoQ10 level and promoted proliferation of fibroblasts. In addition, CoQ10 increased mRNA expression of type I, IV, VII collagen, elastin, and HSP47, whereas CoQ10has little effect on mRNA of type II and VIII MMP. These results suggested that CoQ10 has the efficacy that it increases collagen production in skin, thereby there is possible of the anti-aging by CoQ10 in Japan which reached an aging society, so that it might be based on new physiological function by CoQ10.
Discussion
CoQ10 is essential constituent components in mitochondrial respiratory chain, which play an important role of ATP synthesis and antioxidant effect. In Japan, CoQ10 has been widely commercially available as a dietary and health supplement since 2001 and is used for the prevention of lifestyle-related diseases induced by free radicals and aging. Meanwhile, CoQ10 in the bodies of human beings is thought to be provided by both dietary intake, such as dietary foods and health supplements, and de novo biosynthesis,(8) and CoQ10has a widespread distribution in human tissues, but the abundance of CoQ10 are different between each tissue.(20–22) In particular, CoQ10 levels in skin is very low compared with heart, liver, muscle and brain tissues. Consequently, it is assumed that exogenous CoQ10 play an important role for skin functions.
In this study, intracellular CoQ10 level increased by the addition of 1 or 10 μM P40, and 10 μM P40, and enhanced cell proliferation of skin fibroblasts. A number of studies with using lipid soluble CoQ10 suggested that CoQ10 has anti-aging effect in skin fibroblasts by increased type IV collagen expression and inhibition of UV-induced ROS and MMP-1 production.(9–11) Accordingly, after addition of P40 for 1 week, we confirmed on the expression level of collagen and MMP in fibroblasts by methods of TaqMan with RT-PCR. Like similarly to previous papers, mRNA expression of type IV and VII collagen were elevated by treatment of 1 or 10 μM P40 for 1 week.
Collagen and elastin are important fibrous protein of dermis to maintain normal skin structure and function. Accordingly, decrease of these fibrous proteins are known to be involved in formulation of wrinkle, which are accelerated by UVB and aging. In addition, Inui et al.(10) have reported that collagen is degradated via increasing MMP expression by UVB, and CoQ10 inhibit the upregulation of MMP expression, thus CoQ10 act on collagen to protect in presence of oxidative stress. Moreover, cell proliferation in skin fibroblasts is evoked by addition to CoQ10, so that skin fibroblasts could more produce of collagen and elastin in dermis.
Antioxidants play a critical role in keeping skin healthy. The antioxidant benefits of vitamin C and E are well known, but the importance of the trace mineral, zinc, has been overlooked. This article reviews the evidence supporting zinc's antioxidant role in protecting against free radical-induced oxidative damage. Zinc protects against UV radiation, enhances wound healing, contributes to immune and neuropsychiatric functions, and decreases the relative risk of cancer and cardiovascular disease. All body tissues contain zinc; in skin, it is five to six times more concentrated in the epidermis than the dermis. Zinc is required for the normal growth, development and function of mammals. It is an essential element of more than 200 metalloenzymes, including the antioxidant enzyme, superoxide dismutase, and affects their conformity, stability, and activity. Zinc also is important for the proper functioning of the immune system, and for glandular, reproductive and cell health. Abundant evidence demonstrates the antioxidant role of zinc. Topical zinc, in the form of divalent zinc ions, has been reported to provide antioxidant photoprotection for skin. Two antioxidant mechanisms have been proposed for zinc: zinc ions may replace redox active molecules, such as iron and copper, at critical sites in cell membranes and proteins; alternatively, zinc ions may induce the synthesis of metallothionein, sulfhydryl-rich proteins that protect against free radicals. No matter how they work, topical zinc ions may provide an important and helpful antioxidant defense for skin.
References
- Avila Rodríguez MI, Rodriguez Barroso LG, Sánchez ML. Collagen: A review on its sources and potential cosmetic applications. (https://pubmed.ncbi.nlm.nih.gov/29144022/) J Cosmet Dermatol. 2018 Feb;17(1):20-6. Accessed 5/23/2022.
- Postepy Dermatol Alergol. 2022 Jun; 39(3): 439–445.
Published online 2022 Jul 15. doi: 10.5114/ada.2022.117547
- PMCID: PMC9326919
PMID: 35950117
- Food Sci Nutr. 2023 Jun; 11(6): 2589–2598.
Published online 2023 Mar 23. doi: 10.1002/fsn3.3320
PMCID: PMC10261788
PMID: 37324891
- Front Nutr. 2022; 9: 887113.
Published online 2022 Jun 9. doi: 10.3389/fnut.2022.887113
PMCID: PMC9218663
PMID: 35757262
- Dermatoendocrinol. 2012 Jul 1; 4(3): 253–258.
doi: 10.4161/derm.21923
PMCID: PMC3583886
PMID: 23467280
- Bello AE, Oesser S. Collagen hydrolysate for the treatment of osteoarthritis and other joint disorders: a review of the literature. (https://pubmed.ncbi.nlm.nih.gov/17076983/) Curr Medical Res Opin. 2006 Nov 1;22(11):2221-32. Accessed 5/23/2022.
- Rev Bras Farmacogn. 2023; 33(2): 272–287.
Published online 2023 Feb 7. doi: 10.1007/s43450-023-003701
- PMCID: PMC9904877
PMID: 36778891
- Czajka A, Kania EM, Genovese L, et al. Daily oral supplementation with collagen peptides combined with vitamins and other bioactive compounds improves skin elasticity and has a beneficial effect on joint and general wellbeing. (https://pubmed.ncbi.nlm.nih.gov/30122200/) Nutr Res. 2018 Sep;57:97-108. Accessed 5/23/2022.
- DermNet AZ. Collagen. (https://dermnetnz.org/topics/collagen) Accessed 5/23/2022.
- García-Coronado JM, Martínez-Olvera L, Elizondo-Omaña RE, et al. Effect of collagen supplementation on osteoarthritis symptoms: a meta-analysis of randomized placebo-controlled trials. (https://pubmed.ncbi.nlm.nih.gov/30368550/) Int Orthop. 2019 Mar;43(3):531-538. Accessed 5/23/2022.
- International Food Information Council. Food Insight. What is collagen? (https://foodinsight.org/collagen-supplementation-is-it-all-hype/) Accessed 5/23/2022.
- Association of dietary zinc consumption with periodontitis in diabetes mellitus patients: A cross-sectional study of national health and nutrition examination surveys database (2009-2014).
Xiang M, et al. J Dent Sci. 2024. PMID: 38618104Free PMC article.
Homaeigohar S, et al. ACS Appl Bio Mater. 2023.PMID: 37721636 Free PMC article.
Yazicioglu O, et al. Int Dent J. 2024. PMID: 37709645Free PMC article. Review.
Brecht P, et al. Nutrients. 2023. PMID: 37513682Free PMC article. Review.
Yeh CN, et al. Biol Trace Elem Res. 2023. PMID: 36892689Review.
- Kim DU, Chung HC, Choi J, et al. Oral intake of low-molecular-weight collagen peptide improves hydration, elasticity, and wrinkling in human skin: a randomized, double-blind, placebo-controlled study. (https://pubmed.ncbi.nlm.nih.gov/29949889/) Nutrients. 2018 Jul;10(7):826. Accessed 5/23/2022.
- Lodish H, Berk A, Zipursky SL, et al. Molecular Cell Biology. 4th edition. New York: W. H. Freeman; 2000. Section 22.3, Collagen: The Fibrous Proteins of the Matrix. (https://www.ncbi.nlm.nih.gov/books/NBK21582/) Accessed 5/23/2022.
- Nguyen HP, Katta R. Sugar Sag: Glycation and the Role of Diet in Aging Skin. (https://pubmed.ncbi.nlm.nih.gov/27224842/) Skin Therapy Lett. 2015 Nov;20(6):1-5. Accessed 5/23/2022.
- Andreassen OA, Ferrante RJ, Dedeoglu A, Beal MF. Lipoic acid improves survival in transgenic mouse models of Huntington’s disease. NeuroReport. 2001;12:3371–3373. doi: 10.1097/00001756-200110290-00044. [PubMed] [CrossRef] [Google Scholar]
- Asci H, Erol O, Ellidag HY, Tola EN, Savran M, Ozmen O. Pathology of cigarettes on the reproductive system and ameliorative effects of alpha lipoic acid: a rat model study. Toxicol Ind Health. 2018;34:385–395. doi: 10.1177/0748233718755160. [PubMed] [CrossRef] [Google Scholar]
- Ateyya H, Nader MA, Attia GM, El-Sherbeeny NA. Influence of alpha-lipoic acid on nicotine-induced lung and liver damage in experimental rats. Can J Physiol Pharmacol. 2017;95:492–500. doi: 10.1139/cjpp-2016-0366. [PubMed] [CrossRef] [Google Scholar]
- Attia M, Essa EA, Zaki RM, Elkordy AA. An overview of the antioxidant effects of ascorbic acid and alpha lipoic acid (in liposomal forms) as adjuvant in cancer treatment. Antioxidants. 2020;9:359. doi: 10.3390/antiox9050359. [PMC free article][PubMed] [CrossRef] [Google Scholar]
- Agarwal R, Vasavada N, Sachs NG, Shaw C. Oxidative stress and renal injury with intravenous iron in patients with chronic kidney disease. Kidney Int. 2004;65:2279–2289. doi: 10.1111/j.1523-1755.2004.00648.x.. [PubMed] [CrossRef] [Google Scholar]
- Balercia G, Regoli F, Armeni T, Koverech A, Mantero F, Boscaro M. Placebo-controlled double-blind randomized trial on the use of L-carnitine, L-acetylcarnitine, or combined L-carnitine and L-acetylcarnitine in men with idiopathic asthenozoospermia. Fertil Steril. 2005;84:662–671. doi: 10.1016/j.fertnstert.2005.03.064. [PubMed] [CrossRef] [Google Scholar]
- Bastianetto S, Quirion R. Natural antioxidants and neurodegenerative diseases. Front Biosci Landmark. 2004;9:3447–3452. doi: 10.2741/1493. [PubMed] [CrossRef] [Google Scholar]
- Beer C, Foldbjerg R, Hayashi Y, Sutherland DS, Autrup H. Toxicity of silver nanoparticles — nanoparticle or silver ion ? Toxicol Lett. 2012;208:286–292. doi: 10.1016/j.toxlet.2011.11.002.[PubMed] [CrossRef] [Google Scholar]
- Bingham PM, Stuart SD, Zachar Z. Lipoic acid and lipoic acid analogs in cancer metabolism and chemotherapy. Expert Rev Clin Pharmacol. 2014;7:837–46. doi: 10.1586/17512433.2014.966816.[PubMed] [CrossRef] [Google Scholar]
- Bustamante J, Lodge JK, Marcocci L, Tritschler HJ, Packer L, Rihn BH. α-Lipoic acid in liver metabolism and disease. Free Radical Biol Med. 1998;24:1023–1039. doi: 10.1016/S0891-5849(97)00371-7.[PubMed] [CrossRef] [Google Scholar]
- Brookes MH, Golding BT, Howes DA, Hudson AT. Proof that the absolute configuration of natural α-lipoic acid is R by the synthesis of its enantiomer [(S)-(–)-α-lipoic acid] from (S)-malic acid. J Chem Soc Chem Comm. 1983;19:P1051–1053. doi: 10.1039/C39830001051. [CrossRef] [Google Scholar]
- Buhling KJ, Laakmann E. The effect of micronutrient supplements on male fertility. Curr Opin Obstet Gyn. 2014;26:199–209. doi: 10.1097/GCO.0000000000000063. [PubMed] [CrossRef] [Google Scholar]
- Carrier B, Rideout TC. Anti-obesity and lipid-lowering properties of alpha-lipoic acid. J Hum Nutr Food Sci. 2013;1:1008. [Google Scholar]
- Cavdar Z, Oktan MA, Ural C, Calisir M, Kocak A, Heybeli C, Yildiz S, Arici A, Ellidokuz H, Celik A, Yilmaz O. Renoprotective effects of alpha lipoic acid on iron overload-induced kidney injury in rats by suppressing NADPH oxidase 4 and p38 MAPK signaling. Biol Trace Elem Res. 2020;193:483–493. doi: 10.1007/s12011-019-01733-3. [PubMed] [CrossRef] [Google Scholar]
- Choi HJ, Kim TY, Ruiz-Llorente S, Jeon MJ, Han JM, Kim WG, Shong YK, Kim WB. Alpha-lipoic acid induces sodium iodide symporter expression in TPC-1 thyroid cancer cell line. Nucl Med Biol. 2012;39:1275–1280. doi: 10.1016/j.nucmedbio.2012.08.007. [PubMed] [CrossRef] [Google Scholar]
- Choi K, Kim J, Kim H. α-Lipoic acid treatment is neurorestorative and promotes functional recovery after stroke in rats. J Neurol Sci. 2013;333:e195. doi: 10.1016/j.jns.2013.07.784. [PMC free article][PubMed] [CrossRef] [Google Scholar]
- Choi NH, Kim JG, Yang DJ, Kim YS, Yoo MA. Age-related changes in Drosophila midgut are associated with PVF2, a PDGF/VEGF-like growth factor. Aging Cell. 2008;7:318–334. doi: 10.1111/j.1474-9726.2008.00380.x. [PMC free article] [PubMed] [CrossRef] [Google Scholar]
- Cianci A, Panella M, Fichera M, Falduzzi C, Bartolo M, Caruso S D-chiro-inositol and alpha lipoic acid treatment of metabolic and menses disorders in women with PCOS. Gynecol Endocrinol. 2015;31:483–486. doi: 10.3109/09513590.2015.1014784. [PubMed] [CrossRef] [Google Scholar]
- Cremer DR, Rabeler R, Roberts A, Lynch B. Safety evaluation of α-lipoic acid (ALA) Regul Toxicol Pharm. 2006;46:29–41. doi: 10.1016/j.yrtph.2006.06.004. [PubMed] [CrossRef] [Google Scholar]
- Cui H, Tang D, Garside GB, Zeng T, Wang Y, Tao Z, Zhang L, Tao S. Wnt signaling mediates the aging-induced differentiation impairment of intestinal stem cells. Stem Cell Rev Rep. 2019;15:448–455. doi: 10.1007/s12015-019-09880-9. [PMC free article][PubMed] [CrossRef] [Google Scholar]
- Cure E, Cure MC. Alpha-lipoic acid may protect patients with diabetes against COVID-19 infection. Med Hypotheses. 2020;143:110185. doi: 10.1016/j.mehy.2020.110185. [PMC free article][PubMed] [CrossRef] [Google Scholar]
- De Cicco S, Immediata V, Romualdi D, Policola C, Tropea A, Di Florio C, Tagliaferri V, Scarinci E, Della Casa S, Lanzone A, Apa R. Myoinositol combined with alpha-lipoic acid may improve the clinical and endocrine features of polycystic ovary syndrome through an insulin-independent action. Gynecol Endocrinol. 2017;33:698–701. doi: 10.1080/09513590.2017.1313972. [PubMed] [CrossRef] [Google Scholar]
- Del Rio D, Stewart AJ, Pellegrini N. A review of recent studies on malondialdehyde as toxic molecule and biological marker of oxidative stress. Nutr Metab Cardiovas. 2005;15:P316–328. doi: 10.1016/j.numecd.2005.05.003. [PubMed] [CrossRef] [Google Scholar]
- Di Nicuolo F, D’Ippolito S, Castellani R, Rossi ED, Masciullo V, Specchia M, Mariani M, Pontecorvi A, Scambia G, Di Simone N. Effect of alpha-lipoic acid and myoinositol on endometrial inflammasome from recurrent pregnancy loss women. Am J Reprod Immunol. 2019;82:e13153. doi: 10.1111/aji.13153.[PubMed] [CrossRef] [Google Scholar]
- Di Tucci C, Di Feliciantonio M, Vena F, Capone C, Schiavi MC, Pietrangeli D, Muzii L, Benedetti Panici P. Alpha lipoic acid in obstetrics and gynecology. Gynecol Endocrinol. 2018;34:729–733. doi: 10.1080/09513590.2018.1462320. [PubMed] [CrossRef] [Google Scholar]
- Di Tucci C, Galati G, Mattei G, Bonanni V, Capri O, D’Amelio R, Muzii L, Benedetti Panici P. The role of alpha lipoic acid in female and male infertility: a systematic review. Gynecol Endocrinol. 2021;37:497–505. doi: 10.1080/09513590.2020.1843619. [PubMed] [CrossRef] [Google Scholar]
- Diane A, Mahmoud N, Bensmail I, Khattab N, Abunada HA, Dehbi M. Alpha lipoic acid attenuates ER stress and improves glucose uptake through DNAJB3 cochaperone. Sci Rep. 2020;24:20482. doi: 10.1038/s41598-020-77621-x. [PMC free article][PubMed] [CrossRef] [Google Scholar]
- Dong L, Zhang X, Yang F, Li J, Yu X, Li Y. Effect of oral alpha-lipoic acid (ALA) on the treatment of male infertility: a protocol for systematic review and meta-analysis. Medicine. 2019;98:e18453. doi: 10.1097/MD.0000000000018453. [PMC free article] [PubMed] [CrossRef] [Google Scholar]
- Dozio E, Ruscica M, Passafaro L, Dogliotti G, Steffani L, Pagani A, Demartini G, Esposti D, Fraschini F, Magni P. The natural antioxidant alpha-lipoic acid induces p27Kip1-dependent cell cycle arrest and apoptosis in MCF-7 human breast cancer cells. Eur J Pharmacol. 2010;641:29–34. doi: 10.1016/j.ejphar.2010.05.009. [PubMed] [CrossRef] [Google Scholar]
- Du G, Qiao Y, Zhuo Z, Zhou J, Li X, Liu Z, Li Y, Chen H. Lipoic acid rejuvenates aged intestinal stem cells by preventing age-associated endosome reduction. EMBO Rep. 2020;21:49583. doi: 10.15252/embr.201949583. [PMC free article][PubMed] [CrossRef] [Google Scholar]
- De Jong WH, Borm PJ. Drug delivery and nanoparticles: applications and hazards. Int J Nanomed. 2008;3:133–149. doi: 10.2147/ijn.s596.[PMC free article] [PubMed] [CrossRef] [Google Scholar]
- Del Rio C, Malani PN. COVID-19—new insights on a rapidly changing epidemic. JAMA. 2020;323:1339–1340. doi: 10.1001/jama.2020.3072. [PubMed] [CrossRef] [Google Scholar]
- Dohle G, Jungwirth A, Colpi G, Giwercman A, Diemer T. European Association of Urology Guidelines on Male Infertility: the 2012 update. Eur Urol. 2012;62:324–332. doi: 10.1016/j.eururo.2012.04.048. [PubMed] [CrossRef] [Google Scholar]
- Emir DF, Ozturan IU, Yilmaz S. Alpha lipoic acid intoxicatıon: an adult. Am J Emerg Med. 2018;36:1125–e3. doi: 10.1016/j.ajem.2018.03.022.[PubMed] [CrossRef] [Google Scholar]
- Eremeeva ME, Silverman DJ. Effects of the antioxidant α-lipoic acid on human umbilical vein endothelial cells infected with Rickettsia rickettsii. Infect Immun. 1998;66:2290–2299. doi: 10.1128/IAI.66.5.2290-2299.1998. [PMC free article] [PubMed] [CrossRef] [Google Scholar]
- Freelan RO. The green pigment and physiology of guard cells. Science. 1951;114:94–95. doi: 10.1126/science.114.2952.94. [PubMed] [CrossRef] [Google Scholar]
- Fruzzetti F, Benelli E, Fidecicchi T, Tonacchera M. Clinical and metabolic effects of alpha-lipoic acid associated with two fifferent doses of myo-inositol in women with polycystic ovary syndrome. Int J Endocrinol. 2020;2020:2901393. doi: 10.1155/2020/2901393. [PMC free article][PubMed] [CrossRef] [Google Scholar]
- Fruzzetti F, Capozzi A, Canu A, Lello S. Treatment with D-chiro-inositol and alpha lipoic acid in the management of polycystic ovary syndrome. Gynecol Endocrinol. 2019;35:506–510. doi: 10.1080/09513590.2018.1540573. [PubMed] [CrossRef] [Google Scholar]
- Galeshkalami NS, Abdollahi M, Najafi R, Baeeri M, Jamshidzade A, Falak R, Gholami MD, Hassanzadeh G, Mokhtari T, Hassani S, Rahimifard M. Alpha-lipoic acid and coenzyme Q10 combination ameliorates experimental diabetic neuropathy by modulating oxidative stress and apoptosis. Life Sci. 2018;216:101–110. doi: 10.1016/j.lfs.2018.10.055.[PubMed] [CrossRef] [Google Scholar]
- Genazzani AD, Prati A, Marchini F, Petrillo T, Napolitano A, Simoncini T. Differential insulin response to oral glucose tolerance test (OGTT) in overweight/obese polycystic ovary syndrome patients undergoing to myo-inositol (MYO), alpha lipoic acid (ALA), or combination of both. Gynecol Endocrinol. 2019;35:1088–1093. doi: 10.1080/09513590.2019.1640200. [PubMed] [CrossRef] [Google Scholar]
- Genazzani AD, Shefer K, Della Casa D, Prati A, Napolitano A, Manzo A, Despini G, Simoncini T. Modulatory effects of alpha-lipoic acid (ALA) administration on insulin sensitivity in obese PCOS patients. J Endocrinol Invest. 2018;41:583–590. doi: 10.1007/s40618-017-0782-z. [PubMed] [CrossRef] [Google Scholar]
- Gharagozloo P, Aitken RJ. The role of sperm oxidative stress in male infertility and the significance of oral antioxidant therapy. Hum Reprod. 2011;26:1628–1640. doi: 10.1093/humrep/der132.[PubMed] [CrossRef] [Google Scholar]
- Ghibu S, Richard C, Vergely C, Zeller M, Cottin Y, Rochette L. Antioxidant properties of an endogenous thiol: alpha-lipoic acid, useful in the prevention of cardiovascular diseases. J Cardiovasc Pharmacol. 2009;54:391–398. doi: 10.1097/FJC.0b013e3181be7554. [PubMed] [CrossRef] [Google Scholar]
- Girotti AW, Kriska T. Role of lipid hydroperoxides in photo-oxidative stress signaling. Antioxid Redox Signal. 2004;6:301–310. doi: 10.1089/152308604322899369. [PubMed] [CrossRef] [Google Scholar]
- Golbidi S, Badran M, Laher I. Diabetes and alpha lipoic acid. Front Pharmacol. 2011;2:69. doi: 10.3389/fphar.2011.00069. [PMC free article][PubMed] [CrossRef] [Google Scholar]
- Gomes MB, Negrato CA. Alpha-lipoic acid as a pleiotropic compound with potential therapeutic use in diabetes and other chronic diseases. Diabetol Metab Syndr. 2014;6:80. doi: 10.1186/1758-5996-6-80.[PMC free article] [PubMed] [CrossRef] [Google Scholar]
- Granata S, Dalla Gassa A, Tomei P, Lupo A, Zaza G. Mitochondria: a new therapeutic target in chronic kidney disease. Nutr Metabolism. 2015;12:49. doi: 10.1186/s12986-015-0044-z. [PMC free article][PubMed] [CrossRef] [Google Scholar]
- Guo Z, Lucchetta E, Rafel N, Ohlstein B. Maintenance of the adult Drosophila intestine: all roads lead to homeostasis. Curr Opin Genet Dev. 2006;40:81–86. doi: 10.1016/j.gde.2016.06.009. [PMC free article][PubMed] [CrossRef] [Google Scholar]
- Gupta D, Bhattacharjee A. Detailed investigation of N-(4-n-pentyl-oxybenzylidene)-4′-n-hexylaniline liquid crystal molecule. J Mol Struct. 2019;15:66–77. doi: 10.1016/j.molstruc.2019.06.068. [CrossRef] [Google Scholar]
- Hagen TM, Liu J, Lykkesfeldt J, Wehr CM, Ingersoll RT, Vinarsky V, Bartholomew JC, Ames BN. Feeding acetyl-L-carnitine and lipoic acid to old rats significantly improves metabolic function while decreasing oxidative stress. Proc Natl Acad Sci. 2002;99:1870–1875. doi: 10.1073/pnas.261708898.[PMC free article] [PubMed] [CrossRef] [Google Scholar]
- Huang Y, Zhu Z, Sun M, Wang J, Guo R, Shen L, Wu W. Lipoic acid inhibits cell proliferation of tumor cells in vitro and in vivo. Cancer Biol Ther. 2012;13:1425–1435. doi: 10.4161/cbt.22003. [PMC free article][PubMed] [CrossRef] [Google Scholar]
- Ibrahim SF, Osman K, Das S, Othman AM, Majid NA, Rahman MP. A study of the antioxidant effect of alpha lipoic acids on sperm quality. Clinics. 2008;63:545–550. doi: 10.1590/S1807-59322008000400022. [PMC free article] [PubMed] [CrossRef] [Google Scholar]
- Islam KN, O’Byrne D, Devaraj S, Palmer B, Grundy SM, Jialal I. Alpha-tocopherol supplementation decreases the oxidative susceptibility of LDL in renal failure patients on dialysis therapy. Atherosclerosis. 2000;150:217–224. doi: 10.1016/S0021-9150(99)00410-4. [PubMed] [CrossRef] [Google Scholar]
- Isenmann E, Trittel L, Diel P. The effects of alpha lipoic acid on muscle strength recovery after a single and a short-term chronic supplementation — a study in healthy well-trained individuals after intensive resistance and endurance training. J Int Soc Sports Nutr. 2020;17:61. doi: 10.1186/s12970-020-00389-y.[PMC free article] [PubMed] [CrossRef] [Google Scholar]
- Jiang H, Patel PH, Kohlmaier A, Grenley MO, McEwen DG, Edgar BA. Article JNK activity in somatic stem cells causes loss of tissue homeostasis in the aging Drosophila gut. Cell Stem Cell. 2008;3:442–455. doi: 10.1016/j.stem.2008.07.024.[PMC free article] [PubMed] [CrossRef] [Google Scholar]
- Jibril AT, Jayedi A, Shab-Bidar S (2022) Efficacy and safety of oral alpha-lipoic acid supplementation for type 2 diabetes management: a systematic review and dose–response meta-analysis of randomized trials. Endocr Connect 11. 10.1530/EC-22-0322. [PMC free article] [PubMed]
- Jocelyn PC. The standard redox potential of cysteine-cystine from the thiol-disulphide exchange reaction with glutathione and lipoic acid. Eur J Biochem. 1967;2:327–331. doi: 10.1111/j.1432-1033.1967.tb00142.x. [PubMed] [CrossRef] [Google Scholar]
- Kang SJ, Lee YJ, Lee EK, Kwak MK. Silver nanoparticles-mediated G2/M cycle arrest of renal epithelial cells is associated with NRF2-GSH signaling. Toxicol Lett. 2012;211:334–341. doi: 10.1016/j.toxlet.2012.04.016. [PubMed] [CrossRef] [Google Scholar]
- Kang J, Chong SJ, Ooi VZ, Vali S, Kumar A, Kapoor S, Abbasi T, Hirpara JL, Loh T, Goh BC, Pervaiz S. Overexpression of Bcl-2 induces STAT-3 activation via an increase in mitochondrial superoxide. Oncotarget. 2015;6:34191–34205. doi: 10.18632/oncotarget.5763. [PMC free article][PubMed] [CrossRef] [Google Scholar]
- 1. Piez KA, Trus BL. A new model for packing of type-I collagen molecules in the native fibril. Biosci Rep 1981; 1: 801–810. [PubMed] [Google Scholar]
2. Varani J, Warner RL, Gharaee-Kermani M, et al.Vitamin A antagonizes decreased cell growth and elevated collagen-degrading matrix metalloproteinases and stimulates collagen accumulation in naturally aged human skin. J Invest Dermatol 2000; 114: 480–486. [PubMed] [Google Scholar]
3. Fisher GJ, Quan T, Purohit T, et al. Collagen fragmentation promotes oxidative stress and elevates matrix metalloproteinase-1 in fibroblasts in aged human skin. Am J Pathol 2009; 174: 101–114. [PMC free article] [PubMed] [Google Scholar]
4. Crane FL, Hatefi Y, Lester RL, Widmer C. Isolation of a quinine from beef heart mitochondria. Biochim Biophys Acta 1957; 25: 220–221. [PubMed] [Google Scholar]
5. Bianchi C, Fato R, Genova ML, Parenti Castelli G, Lenaz G. Structural and functional organization of Complex I in the mitochondrial respiratory chain. Biofactors 2003; 18: 3–9. [PubMed] [Google Scholar]
6. Frei B, Kim MC, Ames BN. Ubiquinol-10 is an effective lipid-soluble antioxidant at physiological concentrations. Proc Natl Acad Sci U S A 1990; 87: 4879–4883. [PMC free article] [PubMed] [Google Scholar]
7. Kettawan A, Takahashi T, Kongkachuichai R, Charoenkiatkul S, Kishi T, Okamoto T. Protective effects of coenzyme Q10 on decreased oxidative stress resistance induced by simvastatin. J Clin Biochem Nutr 2007; 40: 194–202. [PMC free article] [PubMed] [Google Scholar]
8. Okamoto T, Fukui K, Nakamoto M, et al. Serum levels of coenzyme Q10 and lipids in patients during total parenteral nutrition. J Nutr Sci Vitaminol (Tokyo) 1986; 32: 1–12. [PubMed] [Google Scholar]
9. Hoppe U, Bergemann J, Diembeck W, et al. Coenzyme Q10, a cutaneous antioxidant and energizer. Biofactors1999; 9: 371–378. [PubMed] [Google Scholar]
10. Inui M, Ooe M, Fujii K, Matsunaka H, Yoshida M, Ichihashi M. Mechanisms of inhibitory effects of CoQ10on UVB-induced wrinkle formation in vitro and in vivo. Biofactors 2008; 32: 237–243. [PubMed] [Google Scholar]
11. Muta-Takada K, Terada T, Yamanishi H, et al.Coenzyme Q10 protects against oxidative stress-induced cell death and enhances the synthesis of basement membrane components in dermal and epidermal cells. Biofactors 2009; 35: 435–441. [PubMed] [Google Scholar]
12. Zhang M, Dang L, Guo F, Wang X, Zhao W, Zhao R. Coenzyme Q10 enhances dermal elastin expression, inhibits IL-1α production and melanin synthesis in vitro. Int J Cosmet Sci 2012; 34: 273–279. [PubMed] [Google Scholar]
13. Nukui K, Yamagishi T, Miyawaki H, Kettawan A, Okamoto T, Sato K. Comparison of uptake between PureSorb-QTM40 and regular hydrophobic coenzyme Q10in rats and humans after single oral intake. J Nutr Sci Vitaminol (Tokyo) 2007; 53: 187–190. [PubMed] [Google Scholar]
14. Okamoto T, Fukunaga Y, Ida Y, Kishi T. Determination of reduced and total ubiquinones in biological materials by liquid chromatography with electrochemical detection. J Chromatogr 1988; 430: 11–19. [PubMed] [Google Scholar]
15. Bradford MM. A rapid and sensitive method for the quantitation of microgram quantities of protein utilizing the principle of protein-dye binding. Anal Biochem 1976; 72: 248–254. [PubMed] [Google Scholar]
16. Mosmann T. Rapid colorimetric assay for cellular growth and survival: application to proliferation and cytotoxicity assays. J Immunol Methods 1983; 65: 55–63. [PubMed] [Google Scholar]
17. Egeblad M, Werb Z. New functions for the matrix metalloproteinases in cancer progression. Nat Rev Cancer 2002; 2: 161–174. [PubMed] [Google Scholar]
18. Ishida Y, Kubota H, Yamamoto A, Kitamura A, Bächinger HP, Nagata K. Type I collagen in Hsp47-null cells is aggregated in endoplasmic reticulum and deficient in N-propeptide processing and fibrillogenesis. Mol Biol Cell 2006; 17: 2346–2355. [PMC free article] [PubMed] [Google Scholar]
19. Philips N, Samuel P, Parakandi H, et al. Beneficial regulation of fibrillar collagens, heat shock protein-47, elastin fiber components, transforming growth factor-β1, vascular endothelial growth factor and oxidative stress effects by copper in dermal fibroblasts. Connect Tissue Res 2012; 53: 373–378. [PubMed] [Google Scholar]
20. Aberg F, Appelkvist EL, Dallner G, Ernster L. Distribution and redox state of ubiquinones in rat and human tissues. Arch Biochem Biophys 1992; 295: 230–234. [PubMed] [Google Scholar]
21. Miles MV, Horn PS, Morrison JA, Tang PH, DeGrauw T, Pesce AJ. Plasma coenzyme Q10 reference intervals, but not redox state, are affected by gender and race in self-reported healthy adults. Clin Chim Acta2003; 332: 123–132. [PubMed] [Google Scholar]
22. Shindo Y, Witt E, Han D, Epstein W, Packer L. Enzymic and non-enzymic antioxidants in epidermis and dermis of human skin. J Invest Dermatol 1994; 102: 122–124. [PubMed] [Google Scholar]
23. Bhagavan HN, Chopra RK, Craft NE, Chitchumroonchokchai C, Failla ML. Assessment of coenzyme Q10 absorption using an in vitro digestion-Caco-2 cell model. Int J Pharm 2007; 333: 112–117. [PubMed] [Google Scholar]
24. Bergamini C, Moruzzi N, Sblendido A, Lenaz G, Fato R. A water soluble CoQ10 formulation improves intracellular distribution and promotes mitochondrial respiration in cultured cells. PLoS One 2012; 7: e33712. [PMC free article] [PubMed] [Google Scholar]
25. Nukui K, Yamagishi T, Miyawaki H, et al. Blood CoQ10 levels and safety profile after singe-dose or chronic administration of PureSorb-QTM40: animal and human studies. Biofactors 2008; 32: 209–219. [PubMed] [Google Scholar]
26. Evans M, Baisley J, Barss S, Guthrie N. A randomized, double-blind trial on the bioavailability of two CoQ10 formulations. J Funct Foods 2009; 1: 65–73. [Google Scholar]
27. Kalén A, Appelkvist EL, Dallner G. Age-related changes in the lipid compositions of rat and human tissue. Lipids 1989; 24: 579–584. [PubMed] [Google Scholar]
28. Koide T. Designed triple-helical peptides as tools for collagen biochemistry and matrix engineering. Philos Trans R Soc Lond Biol Sci 2007; 362: 1281–1291. [PMC free article] [PubMed] [Google Scholar]
- Karunakaran S, Diwakar L, Saeed U, Agarwal V, Ramakrishnan S, Iyengar S, Ravindranath V. Activation of apoptosis signal regulating kinase 1 (ASK1) and translocation of death-associated protein, Daxx, in substantia nigra pars compacta in a mouse model of Parkinson’s disease: protection by α-lipoic acid. FASEB J. 2007;21:2226–2236. doi: 10.1096/fj.06-7580com. [PubMed] [CrossRef] [Google Scholar]
- Keith DJ, Butler JA, Bemer B, Dixon B, Johnson S, Garrard M, Sudakin DL, Christensen JM, Pereira C, Hagen TM. Age and gender dependent bioavailability of R-and R, S-α-lipoic acid: a pilot study. Pharmacol Res. 2012;66:199–206. doi: 10.1016/j.phrs.2012.05.002. [PMC free article][PubMed] [CrossRef] [Google Scholar]
- Kothari IR, Mazumdar S, Sharma S, Italiya K, Mittal A, Chitkara D. Docetaxel and alpha-lipoic acid co-loaded nanoparticles for cancer therapy. Ther Deliv. 2019;10:227–240. doi: 10.4155/tde-2018-0074.[PubMed] [CrossRef] [Google Scholar]
- Kumar P, Kadakol A, Krishna Shasthrula P, Arunrao Mundhe N, Sudhir Jamdade V, Barua C, C, Bhanudas Gaikwad A, Curcumin as an adjuvant to breast cancer treatment. Anticancer Agents Med Chem. 2015;15:647–656. doi: 10.2174/1871520615666150101125918.[PubMed] [CrossRef] [Google Scholar]
- Lai S, Petramala L, Muscaritoli M, Cianci R, Mazzaferro S, Mitterhofer AP, Pasquali M, D’Ambrosio V, Carta M, Ansuini M, Ramaccini C. α-Lipoic acid in patients with autosomal dominant polycystic kidney disease. Nutrition. 2020;71:110594. doi: 10.1016/j.nut.2019.110594. [PubMed] [CrossRef] [Google Scholar]
- Langston JW. Mechanism of MPTP toxicity: more answers, more questions. Trends Pharmacol Sci. 1985;6:375–378. doi: 10.1016/0165-6147(85)90176-2. [CrossRef] [Google Scholar]
- Lee SJ, Kang JG, Ryu OH, Kim CS, Ihm SH, Choi MG, Yoo HJ, Kim DS, Kim TW. Effects of α-lipoic acid on transforming growth factor β1-p38 mitogen-activated protein kinase-fibronectin pathway in diabetic nephropathy. Metabolism. 2009;58:616–623. doi: 10.1016/j.metabol.2008.12.006. [PubMed] [CrossRef] [Google Scholar]
- Leehey DJ, Palubiak DJ, Chebrolu S, Agarwal R. Sodium ferric gluconate causes oxidative stress but not acute renal injury in patients with chronic kidney disease: a pilot study. Nephrol Dial Transpl. 2005;20:35–140. doi: 10.1093/ndt/gfh565. [PubMed] [CrossRef] [Google Scholar]
- Levey AS, Beto JA, Coronado BE, Eknoyan G, Foley RN, Kasiske BL, Klag MJ, Mailloux LU, Manske CL, Meyer KB, Parfrey PS. Controlling the epidemic of cardiovascular disease in chronic renal disease: what do we know? What do we need to learn? Where do we go from here? National Kidney Foundation Task Force on Cardiovascular Disease. Am J Kidney Dis. 1998;32:853–906. doi: 10.1016/s0272-6386(98)70145-3. [PubMed] [CrossRef] [Google Scholar]
- Li DW, Wang YD, Zhou SY, Sun WP. α-Lipoic acid exerts neuroprotective effects on neuronal cells by upregulating the expression of PCNA via the P53 pathway in neurodegenerative conditions. Mol Med Rep. 2016;14:4360–4366. doi: 10.3892/mmr.2016.5754. [PubMed] [CrossRef] [Google Scholar]
- Li Y, Ma QG, Zhao LH, Guo YQ, Duan GX, Zhang JY, Ji C. Protective efficacy of alpha-lipoic acid against aflatoxinB1-induced oxidative damage in the liver. Asian-Australas J Anim Sci. 2014;27:907–915. doi: 10.5713/ajas.2013.13588. [PMC free article][PubMed] [CrossRef] [Google Scholar]
- Lim PS, Wei YH, Yu YL, Kho B. Enhanced oxidative stress in haemodialysis patients receiving intravenous iron therapy. Nephrol Dial Transpl. 1999;14:2680–2687. doi: 10.1093/ndt/14.11.2680. [PubMed] [CrossRef] [Google Scholar]
- Liu W, Shi LJ, Li SG. The immunomodulatory effect of alpha-lipoic acid in autoimmune diseases. BioMed Res Int. 2019;2019:8086257. doi: 10.1155/2019/8086257. [PMC free article][PubMed] [CrossRef] [Google Scholar]
- Liu Z, Guo J, Sun H, Huang Y, Zhao R, Yang X. Biochimie α-lipoic acid attenuates LPS-induced liver injury by improving mitochondrial function in association with GR mitochondrial DNA occupancy. Biochimie. 2015;116:52–60. doi: 10.1016/j.biochi.2015.06.023. [PubMed] [CrossRef] [Google Scholar]
- Lyseng-Williamson KA, Fenton C. Docetaxel Drugs. 2005;65:2513–2531. doi: 10.2165/00003495-200565170-00007. [PubMed] [CrossRef] [Google Scholar]
- Maldonado-Rojas W, Olivero-Verbel J, Ortega-Zuñiga C. Searching of protein targets for alpha lipoic acid. J Braz Chem Soc. 2011;22:2250–2259. doi: 10.1590/S0103-50532011001200003. [CrossRef] [Google Scholar]
- Malgras B, Berger A, Bazeries P, Aubé C, Boudiaf M, Soyer P (2016) Imaging of complications of colonic stents. In: Tonolini, M. (eds) Imaging complications of gastrointestinal and biliopancreatic endoscopy procedures. Springer, Cham. 10.1007/978-3-319-31211-8_14.
- Marangon K, Devaraj S, Tirosh O, Packer L, Jialal I. Comparison of the effect of α-lipoic acid and α-tocopherol supplementation on measures of oxidative stress. Free Radic Biol Med. 1999;27:1114–1121. doi: 10.1016/S0891-5849(99)00155-0. [PubMed] [CrossRef] [Google Scholar]
- Metwaly HH, Fathy SA, Abdel Moneim MM, Emam MA, Soliman AF, El-Naggar ME, Omara EA, El-Bana MA. Chitosan and solid lipid nanoparticles enhance the efficiency of alpha-lipoic acid against experimental neurotoxicity. Toxicol Mech Methods. 2022;32:268–279. doi: 10.1080/15376516.2021.1998275. [PubMed] [CrossRef] [Google Scholar]
- Moffa S, Improta I, Rocchetti S, Mezza T, Giaccari A. Potential cause-effect relationship between insulin autoimmune syndrome and alpha lipoic acid: two case reports. Nutrition. 2019;57:1–4. doi: 10.1016/j.nut.2018.04.010. [PubMed] [CrossRef] [Google Scholar]
- Moon H. Chemopreventive effects of alpha lipoic acid on obesity-related cancers. Ann Nutr Metab. 2016;68:137–44. doi: 10.1159/000443994. [PubMed] [CrossRef] [Google Scholar]
- Moura FA, Andrade KQ, Santos JCF, Goulart MOF (2021) Lipoic acid: its antioxidant and anti-inflammatory role and clinical applications 15:458-483. 10.2174/1568026615666150114161358 [PubMed]
- Na MH, Seo EY, Kim WK. Effects of α-lipoic acid on cell proliferation and apoptosis in MDA-MB-231 human breast cells. Nutr Res Pract. 2009;3:265–271. doi: 10.4162/nrp.2009.3.4.265. [PMC free article][PubMed] [CrossRef] [Google Scholar]
- Namazi N, Larijani B, Azadbakht L. Alpha-lipoic acid supplement in obesity treatment: a systematic review and meta-analysis of clinical trials. Clin Nutr. 2017;37:419–428. doi: 10.1016/j.clnu.2017.06.002.[PubMed] [CrossRef] [Google Scholar]
- Omran OM, Omer OH. The effects of alpha-lipoic acid on breast of female albino rats exposed to malathion: histopathological and immunohistochemical study. Pathol Res Pract. 2015;211:462–469. doi: 10.1016/j.prp.2015.02.006.[PubMed] [CrossRef] [Google Scholar]
- Ou P, Tritschler HJ, Wolff SP. Thioctic (lipoic) acid: a therapeutic metal-chelating antioxidant? Biochem Pharmacol. 1995;50:123–126. doi: 10.1016/0006-2952(95)00116-H. [PubMed] [CrossRef] [Google Scholar]
- Packer L, Witt EH, Tritschler HJ. Alpha-lipoic acid as a biological antioxidant. Free Radical Biol Med. 1995;19:227–250. doi: 10.1016/0891-5849(95)00017-R. [PubMed] [CrossRef] [Google Scholar]
- Park S, Karunakaran U, Ho Jeoung N, Jeon JH, Lee IK. Physiological effect and therapeutic application of alpha lipoic acid. Curr Med Chem. 2014;21:3636–45. doi: 10.2174/0929867321666140706141806.[PubMed] [CrossRef] [Google Scholar]
- Phulara SC, Pandey S, Jha A, Chauhan PS, Gupta P, Shukla V. Hemiterpene compound, 3,3-dimethylallyl alcohol promotes longevity and neuroprotection in Caenorhabditis elegans. Geroscience. 2021;43:791–807. doi: 10.1007/s11357-020-00241-w. [PMC free article] [PubMed] [CrossRef] [Google Scholar]
- Rezaie M, Nasehi M, Vaseghi S, Mohammadi-Mahdiabadi-Hasani MH, Zarrindast MR, Nasiri Khalili MA. The protective effect of alpha lipoic acid (ALA) on social interaction memory, but not passive avoidance in sleep-deprived rats. N-S Arch Pharmacol. 2020;393:2081–2091. doi: 10.1007/s00210-020-01916-z. [PubMed] [CrossRef] [Google Scholar]
- Rogers SA. Lipoic acid as a potential first agent for protection from mycotoxins and treatment of mycotoxicosis. Arch Environ Health. 2003;58:528–532. doi: 10.3200/AEOH.58.8.528-532. [PubMed] [CrossRef] [Google Scholar]
- Roob JM, Khoschsorur G, Tiran A, Horina JH, Holzer H, Winklhofer-Roob BM. Vitamin E attenuates oxidative stress induced by intravenous iron in patients on hemodialysis. J Am Soc Nephrol. 2000;11:539–549. doi: 10.1681/ASN.V113539. [PubMed] [CrossRef] [Google Scholar]
- Rousseau AS, Sibille B, Murdaca J, Mothe-Satney I, Grimaldi PA, Neels JG. Α-lipoic acid up-regulates expression of peroxisome proliferator-activated receptor b in skeletal muscle: involvement of the JNK signaling pathway. FASEB J. 2016;30:1287–1299. doi: 10.1096/fj.15-280453. [PubMed] [CrossRef] [Google Scholar]
- Sacks D, Baxter B, Campbell BC, Carpenter JS, Cognard C, Dippel D, Eesa M, Fischer U, Hausegger K, Hirsch JA. Multisociety consensus quality improvement revised consensus statement for endovascular therapy of acute ischemic stroke. Int J Stroke. 2018;13:612–632. doi: 10.1177/1747493018778713. [PubMed] [CrossRef] [Google Scholar]
- Sahni A, Fang R, Sahni SK, Walker DH. Pathogenesis of Rickettsial diseases: pathogenic and immune mechanisms of an endotheliotropic infection. Annu Rev Pathol. 2019;14:127–152. doi: 10.1146/annurev-pathmechdis-012418-012800. [PMC free article][PubMed] [CrossRef] [Google Scholar]
- Salehi B, Berkay Yılmaz Y, Antika G, Boyunegmez Tumer T, Fawzi Mahomoodally M, Lobine D, Akram M, Riaz M, Capanoglu E, Sharopov F, Martins N. Insights on the use of α-lipoic acid for therapeutic purposes. Biomolecules. 2019;9:356. doi: 10.3390/biom9080356. [PMC free article][PubMed] [CrossRef] [Google Scholar]
- Sayıner S, Serakıncı N. Alpha-lipoic acid as a potential treatment for COVID-19 — a hypothesis. Curr Top Nutraceutical Res. 2021;19:172–175. doi: 10.37290/ctnr2641-452x.19:172-175. [CrossRef] [Google Scholar]
- Schoen K, Horvat N, Guerreiro NF, de Castro I, de Giassi KS. Spectrum of clinical and radiographic findings in patients with diagnosis of H1N1 and correlation with clinical severity. BMC Infec Dis. 2019;19:964. doi: 10.1186/s12879-019-4592-0. [PMC free article] [PubMed] [CrossRef] [Google Scholar]
- Schultz MB, Sinclair DA. When stem cells grow old: phenotypes and mechanisms of stem cell aging. Development. 2016;143:3–14. doi: 10.1242/dev.130633. [PMC free article][PubMed] [CrossRef] [Google Scholar]
- Searls RL, Sanadi DR. α-Ketoglutaric dehydrogenase: VIII. Isolation and some properties of a flavoprotein component. J Biol Chem. 1960;235:2485–2491. doi: 10.1016/S0021-9258(18)64646-0. [PubMed] [CrossRef] [Google Scholar]
- Shay KP, Moreau RF, Smith EJ, Smith AR, Hagen TM. Alpha-lipoic acid as a dietary supplement: molecular mechanisms and therapeutic potential. BBA-General Subjects. 2009;1790:1149–1160. doi: 10.1016/j.bbagen.2009.07.026. [PMC free article][PubMed] [CrossRef] [Google Scholar]
- Sagi-Dain L, Sagi S, Dirnfeld M. The effect of paternal age on oocyte donation outcomes. Obstet Gynecol Surv. 2017;71:301–306. doi: 10.1097/01.pec.0000526609.89886.37. [PubMed] [CrossRef] [Google Scholar]
- Shinto L, Quinn J, Montine T, Dodge HH, Woodward W, Baldauf-Wagner S, Waichunas D, Bumgarner L, Bourdette D, Silbert L, Kaye J. A randomized placebo-controlled pilot trial of omega-3 fatty acids and alpha lipoic acid in Alzheimer’s disease. J Alzheimer Dis. 2014;38:111–120. doi: 10.3233/JAD-130722. [PMC free article] [PubMed] [CrossRef] [Google Scholar]
- Sola S, Mir MQ, Cheema FA, Khan-Merchant N, Menon RG, Parthasarathy S, Khan BV. Irbesartan and lipoic acid improve endothelial function and reduce markers of inflammation in the metabolic syndrome: results of the Irbesartan and Lipoic Acid in Endothelial Dysfunction (ISLAND) study. Circulation. 2005;111:343–349. doi: 10.1161/01.CIR.0000153272.48711.B9.[PubMed] [CrossRef] [Google Scholar]
- Solmonson A, DeBerardinis RJ. Lipoic acid metabolism and mitochondrial redox regulation. J Biol Chem. 2018;293:7522–7530. doi: 10.1074/jbc.TM117.000259. [PMC free article][PubMed] [CrossRef] [Google Scholar]
- Song Z, Xu Y, Bao L, Zhang L, Yu P, Qu Y, Zhu H, Zhao W, Han Y, Qin C. From SARS to MERS, thrusting coronaviruses into the spotlight. Viruses. 2019;11:59. doi: 10.3390/v11010059. [PMC free article] [PubMed] [CrossRef] [Google Scholar]
- Suh JH, Shenvi SV, Dixon BM, Liu H, Jaiswal AK, Liu RM, Hagen TM. Decline in transcriptional activity of Nrf2 causes age-related loss of glutathione synthesis, which is reversible with lipoic acid. Proc Natl Acad Sci US A. 2004;101:3381–3386. doi: 10.1073/pnas.0400282101. [PMC free article][PubMed] [CrossRef] [Google Scholar]
- Takei GL, Mukai C, Okuno M. Transient Ca 2 + mobilization caused by osmotic shock initiates salmonid fish sperm motility. J Exp Biol. 2012;15:630–641. doi: 10.1242/jeb.063628. [PubMed] [CrossRef] [Google Scholar]
- Teichret J, Hermann R, Ruus P, Preiss R. Plasma kinetics, metabolism, and urinary excretion of alpha-lipoic acid following oral administration in healthy volunteers. J Clin Pharmacol. 2003;43:1257–1267. doi: 10.1177/0091270003258654. [PubMed] [CrossRef] [Google Scholar]
- Thuwanut P, Chatdarong K, Johannisson A, Bergqvist AS, Söderquist L, Axnér E. Cryopreservation of epididymal cat spermatozoa: effects of in vitroantioxidative enzymes supplementation and lipid peroxidation induction. Theriogenology. 2010;73:1076–1087. doi: 10.1016/j.theriogenology.2010.01.007. [PubMed] [CrossRef] [Google Scholar]
- Tripathi AK, Ray AK, Mishra SK. Molecular and pharmacological aspects of piperine as a potential molecule for disease prevention and management: evidence from clinical trials. Beni-Suef Univ J Basic Appl Sci. 2022;11:16. doi: 10.1186/s43088-022-00196-1. [PMC free article] [PubMed] [CrossRef] [Google Scholar]
- Tripathy J, Tripathy A, Thangaraju M, Suar M, Elangovan S. α-Lipoic acid inhibits the migration and invasion of breast cancer cells through inhibition of TGFβ signaling. Life Sci. 2018;207:15–22. doi: 10.1016/j.lfs.2018.05.039. [PubMed] [CrossRef] [Google Scholar]
- Trivedi P, Jena GB. Role of α-lipoic acid in dextran sulfate sodium-induced ulcerative colitis in mice: studies on inflammation, oxidative stress, DNA damage and fibrosis. Food Chem Toxicol. 2013;59:339–355. doi: 10.1016/j.fct.2013.06.019.[PubMed] [CrossRef] [Google Scholar]
- Verma SK, Jha E, Panda PK, Mishra A, Thirumurugan A, Das B, Parashar SK, Suar M. Rapid novel facile biosynthesized silver nanoparticles from bacterial release induce biogenicity and concentration dependent in vivo cytotoxicity with embryonic zebrafish—a mechanistic insight. Toxicol Sci. 2018;161:125–138. doi: 10.1093/toxsci/kfx204.[PubMed] [CrossRef] [Google Scholar]
- Vigil M, Berkson BM, Garcia AP. Adverse effects of high doses of intravenous alpha lipoic acid on liver mitochondria. Glob Adv Health Med. 2014;3:25–27. doi: 10.7453/gahmj.2013.011. [PMC free article][PubMed] [CrossRef] [Google Scholar]
- Wang Q, Lv C, Sun Y, Han X, Wang S, Mao Z, Xin Y, Zhang B. The role of alpha-lipoic acid in the pathomechanism of acute ischemic stroke. Cell Physiol Biochem. 2018;48:42–53. doi: 10.1159/000491661. [PubMed] [CrossRef] [Google Scholar]
- Wenzel U, Nickel A, Daniel H. α-Lipoic acid induces apoptosis in human colon cancer cells by increasing mitochondrial respiration with a concomitant O2−.-generation. Apoptosis. 2005;10:359–368. doi: 10.1007/s10495-005-0810-x. [PubMed] [CrossRef] [Google Scholar]
- Wollin SD, Jones PJ. α-Lipoic acid and cardiovascular disease. J Nutr. 2003;133:3327–3330. doi: 10.1093/jn/133.11.3327. [PubMed] [CrossRef] [Google Scholar]
- Xiong Y, Li Q, Ding Z, Zheng J, Zhou D, Wei S, Han X, Cheng X, Li X, Xue Y (2022) Dietary α-lipoic acid requirement and its effects on antioxidant status, carbohydrate metabolism, and intestinal microflora in Oriental River prawn Macrobrachium nipponense (De Haan). aquaculture 547:737531. 10.1016/j.aquaculture.2021.737531.
- Yadav S, Dwivedi A, Tripathi A, Tripathi AK. Therapeutic potential of short-chain fatty acid production by gut microbiota in neurodegenerative disorders. Nutr Res. 2022;106:72–84. doi: 10.1016/j.nutres.2022.07.007. [PubMed] [CrossRef] [Google Scholar]
- Yadav N, Tripathi AK, Parveen A. PLGA-quercetin nano-formulation inhibits cancer progression via mitochondrial dependent caspase-3, 7 and independent FoxO1 activation with concomitant PI3K/AKT suppression. Pharmaceutics. 2022;14:1326. doi: 10.3390/pharmaceutics14071326. [PMC free article] [PubMed] [CrossRef] [Google Scholar]
- Yan T, Zhang Z, Li D. NGF receptors and PI3K/AKT pathway involved in glucose fluctuation-induced damage to neurons and α-lipoic acid treatment. BMC Neurosci. 2020;21:38. doi: 10.1186/s12868-020-00588-y. [PMC free article] [PubMed] [CrossRef] [Google Scholar]
- Yang JK, Feng Y, Yuan MY, Yuan SY, Fu HJ, Wu BY, Sun GZ, Yang GR, Zhang XL, Wang L, Xu X. Plasma glucose levels and diabetes are independent predictors for mortality and morbidity in patients with SARS. Diabet Med. 2006;23:623–628. doi: 10.1111/j.1464-5491.2006.01861.x. [PubMed] [CrossRef] [Google Scholar]
- Yıldırım Baş F, Bayram D, Arslan B, Armağan I, Yeşilot Ş, Çiçek E, Yorgancıgil E. Effect of alpha lipoic acid on smoking-induced skin damage. Cutan Ocul Toxicol. 2017;36:67–73. doi: 10.3109/15569527.2016.1154069. [PubMed] [CrossRef] [Google Scholar]
- Zhao G, Hu C, Xue Y. In vitro evaluation of chitosan-coated liposome containing both coenzyme Q10 and alpha-lipoic acid: cytotoxicity, antioxidant activity, and antimicrobial activity. J Cosmet Dermatol. 2018;17:258–262. doi: 10.1111/jocd.12369. [PubMed] [CrossRef] [Google Scholar]
- Zhang J, McCullough PA. Lipoic acid in the prevention of acute kidney injury. Nephron. 2016;134:133–140. doi: 10.1159/000448666.[PubMed] [CrossRef] [Google Scholar]
- Ziegler D, Low PA, Freeman R, Tritschler H, Vinik AI. Predictors of improvement and progression of diabetic polyneuropathy following treatment with α-lipoic acid for 4 years in the NATHAN 1 trial. J Diabetes Complications. 2016;30:350–356. doi: 10.1016/j.jdiacomp.2015.10.018. [PubMed] [CrossRef] [Google Scholar]
- Ricard-Blum S. The collagen family. (https://pubmed.ncbi.nlm.nih.gov/21421911/) Cold Spring Harb Perspect Biol. 2011;3(1):a004978. Published 2011 Jan 1. Accessed 5/23/2022.
- Sangsuwan W, Asawanonda P. Four-weeks daily intake of oral collagen hydrolysate results in improved skin elasticity, especially in sun-exposed areas: a randomized, double-blind, placebo-controlled trial. (https://pubmed.ncbi.nlm.nih.gov/32009486/) J Dermatolog Treat. 2020 Mar 9:1-6. Accessed 5/23/2022.
- The Skin Cancer Foundation. Sun & Skin News. Photoaging: What you need to know about the other kind of aging. (https://www.skincancer.org/blog/photoaging-what-you-need-to-know/) Accessed 5/23/2022.
- U.S. National Library of Medicine. MedlinePlus. Collagen Peptides. (https://medlineplus.gov/druginfo/natural/1606.html#Action) Accessed 5/23/2022.
- Wu M, Cronin K, Crane JS. Biochemistry, Collagen Synthesis. (https://www.ncbi.nlm.nih.gov/books/NBK507709/) [Updated 2020 Sep 11]. In: StatPearls [Internet]. Treasure Island (FL): StatPearls Publishing; 2021 Jan-. Accessed 5/23/2022.
- Oliver RF, Barker H, Cooke A, Grant RA (January 1982). "Dermal collagen implants". Biomaterials. 3 (1): 38–40. doi:10.1016/0142-9612(82)90059-X. PMID 7066465.
- Braun R.K., Molitor-Dart M., Wigfield C., Xiang Z., Fain S.B., Jankowska-Gan E., Seroogy C.M., Burlingham W.J., Wilkes D.S., Brand D.D., et al. Transfer of tolerance to collagen type v suppresses T-helper-cell-17 lymphocyte-mediated acute lung transplant rejection. Transplantation. 2009;88:1341–1348. doi: 10.1097/TP.0b013e3181bcde7b. [PMC free article] [PubMed] [CrossRef] [Google Scholar]
- D.J. Leeming, M.A. Karsdal, in Biochemistry of Collagens, Laminins and Elastin (Second Edition), 2019
- K. Henriksen, M.A. Karsdal, in Biochemistry of Collagens, Laminins and Elastin (Second Edition), 2019
- G Shen. Orthod Craniofac Res. 2005 Feb.
- Jocelyn Ross Witstein, MD, FAAOS
- Amy Myers, MD
- MDPI and ACS Style
Cánovas, F.; Abellán-Ruíz, M.S.; García-Muñoz, A.M.; Luque-Rubia, A.J.; Victoria-Montesinos, D.; Pérez-Piñero, S.; Sánchez-Macarro, M.; López-Román, F.J. Randomised Clinical Trial to Analyse the Efficacy of Eggshell Membrane to Improve Joint Functionality in Knee Osteoarthritis. Nutrients 2022, 14, 2340. https://doi.org/10.3390/nu14112340
AMA Style
Cánovas F, Abellán-Ruíz MS, García-Muñoz AM, Luque-Rubia AJ, Victoria-Montesinos D, Pérez-Piñero S, Sánchez-Macarro M, López-Román FJ. Randomised Clinical Trial to Analyse the Efficacy of Eggshell Membrane to Improve Joint Functionality in Knee Osteoarthritis. Nutrients. 2022; 14(11):2340. https://doi.org/10.3390/nu14112340
Chicago/Turabian Style
- Cánovas, Fernando, María Salud Abellán-Ruíz, Ana María García-Muñoz, Antonio Jesús Luque-Rubia, Desirée Victoria-Montesinos, Silvia Pérez-Piñero, Maravilla Sánchez-Macarro, and Francisco Javier López-Román. 2022. "Randomised Clinical Trial to Analyse the Efficacy of Eggshell Membrane to Improve Joint Functionality in Knee Osteoarthritis" Nutrients 14, no. 11: 2340. https://doi.org/10.3390/nu14112340